Click here for information on how to simulate and analyze
Frame Relay traffic.
View this file in pdf
format.
Frame Relay is a protocol standard for
LAN internetworking which provides a fast and efficient method of
transmitting information from a user device to LAN bridges and
routers.
The Frame Relay protocol uses a frame
structured similar to that of LAPD, except that the frame header is
replaced by a 2-byte Frame Relay header field. The Frame Relay
header contains the user-specified DLCI field, which is the
destination address of the frame. It also contains congestion and
status signals which the network sends to the user.
The Frame Relay frame is transmitted to
its destination by way of virtual circuits (logical paths from an
originating point in the network) to a destination point. Virtual
circuits may be permanent (PVCs) or switched (SVCs). PVCs are set up
administratively by the network manager for a dedicated
point-to-point connection; SVCs are set up on a call-by-call
basis.
Frame Relay offers an attractive
alternative to both dedicated lines and X.25 networks for connecting
LANs to bridges and routers. The success of the Frame Relay protocol
is based on the following two underlying factors:
- Because virtual circuits consume
bandwidth only when they transport data, many virtual circuits can
exist simultaneously across a given transmission line. In
addition, each device can use more of the bandwidth as necessary,
and thus operate at higher speeds.
- The improved reliability of
communication lines and increased error-handling sophistication at
end stations allows the Frame Relay protocol to discard erroneous
frames and thus eliminate time-consuming error-handling
processing.
These two factors make Frame Relay a
desirable choice for data transmission; however, they also
necessitate testing to determine that the system works properly and
that data is not lost.
Frame Relay Structure
Standards for the Frame Relay protocol
have been developed by ANSI and CCITT simultaneously. The separate
LMI specification has basically been incorporated into the ANSI
specification. The following discussion of the protocol structure
includes the major points from these specifications.
The Frame Relay frame structure is based
on the LAPD protocol. In the Frame Relay structure, the frame header
is altered slightly to contain the Data Link Connection Identifier
(DLCI) and congestion bits, in place of the normal address and
control fields. This new Frame Relay header is 2 bytes in length and
has the following format:
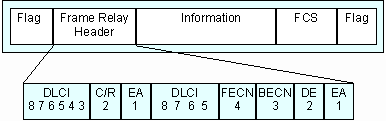
Frame Relay header structure
Explicit Congestion
Notification (ECN) Bits
When the network becomes congested to
the point that it cannot process new data transmissions, it begins
to discard frames. These discarded frames are retransmitted, thus
causing more congestion. In an effort to prevent this situation,
several mechanisms have been developed to notify user devices at the
onset of congestion, so that the offered load may be
reduced.
Two bits in the Frame Relay header are
used to signal the user device that congestion is occurring on the
line: They are the Forward Explicit Congestion Notification (FECN)
bit and the Backward Explicit Congestion Notification (BECN) bit.
The FECN is changed to 1 as a frame is sent downstream toward the
destination location when congestion occurs during data
transmission. In this way, all downstream nodes and the attached
user device learn about congestion on the line. The BECN is changed
to 1 in a frame traveling back toward the source of data
transmission on a path where congestion is occurring. Thus the
source node is notified to slow down transmission until congestion
subsides.
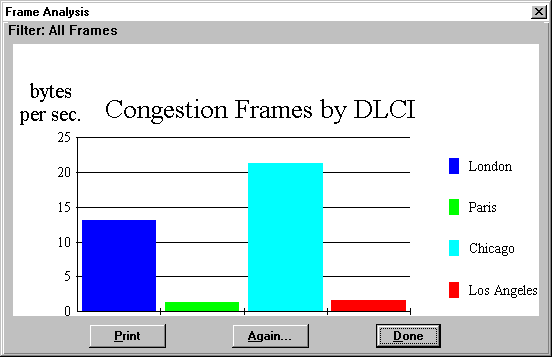
Bytes with congestion bits set according to DLCI
value
It may occur that there are no frames
traveling back to the source node which is causing the congestion.
In this case, the network will want to send its own message to the
problematic source node. The standard, however, does not allow the
network to send its own frames with the DLCI of the desired virtual
circuit.
To address this problem, ANSI defined
the Consolidated Link Layer Management (CLLM). With CLLM, a separate
DLCI (number 1023) is reserved for sending link layer control
messages from the network to the user device. The ANSI standard
(T1.618) defines the format of the CLLM message. It contains a code
for the cause of the congestion and a listing of all DLCIs that
should act to reduce their data transmission to lower
congestion.
Each DLCI corresponds to a PVC
(Permanent Virtual Circuit). It is sometimes necessary to transmit
information about this connection (e.g., whether the interface is
still active) the valid DLCIs for the interface and the status of
each PVC. This information is transmitted using the reserved DLCI
1023 or DLCI 0, depending on the standard used.
A multicast status may also be sent with
the LMI. Multicasting is where a router sends a frame on a reserved
DLCI known as a multicast group. The network then replicates the
frame and delivers it to a predefined list of DLCIs, thus
broadcasting a single frame to a collection of destinations.
When there is congestion on the line,
the network must decide which frames to discard in order to free the
line. Discard Eligibility provides the network with a signal to
determine which frames to discard. The network will discard frames
with a DE value of 1 before discarding other frames.
The DE bit may be set by the user on
some of its lower-priority frames. Alternatively, the network may
set the DE bit to indicate to other nodes that a frame should be
preferentially selected for discard, if necessary.
Frame Relay
Standards
T1.618 describes the protocol supporting
the data transfer phase of the Frame Relay bearer service, as
defined in ANSI T1.606. T1.618 is based on a subset of ANSI T1.602
(LAPD) called the "Core Aspects" and is used by both switched and
permanent virtual calls.
T1.618 also includes the Consolidated
Link Layer Mechanism (CLLM). The generation and transport of CLLM is
optional. With CLLM, DLCI 1023 is reserved for sending link layer
control messages.
T1.618 issues implicit congestion
notification from the network to the user device. The congestion
notification contains a code indicating the cause of the congestion
and lists all DLCIs that have to reduce their traffic in order to
lower congestion.
To establish a Switched Virtual Circuit
(SVC) connection, Frame Relay users must establish a dialog with the
network using the signalling specification in T1.617. The procedure
results in the assignment of a DLCI. Once the dialog is established,
the T1.618 procedures apply.
To establish a Permanent Virtual Circuit
(PVC), a setup protocol is used which is identical to the ISDN
D-channel protocol and defined in T1.617.
With ISDN, users can use the D-channel
for setup. For non-ISDN callers, there is no D-channel, so the
dialog between the user and the network must be separated from the
ordinary data transfer procedures. In T1.617, DLCI 0 is
reserved.
T1.617 also contains specifications on
how Frame Relay service parameters are negotiated.
ANSI LMI is a Permanent Virtual Circuit
(PVC) management system defined in Annex D of T1.617. ANSI LMI is
virtually identical to the Manufacturers’ LMI, without the optional
extensions. ANSI LMI uses DLCI 0.
Manufacturers’ LMI is a Frame Relay
specification with extension-document number 001-208966, September
18, 1990.
Manufacturers’ LMI defines a generic
Frame Relay service based on PVCs for interconnecting DTE devices
with Frame Relay network equipment. In addition to the ANSI
standard, Manufacturers’ LMI includes extensions and LMI functions
and procedures. Manufacturers’ LMI uses DLCI 1023.
Your analyzer also supports the decoding
of Network-to-Network (NNI) PVC frames according to the Frame Relay
Forum implementation agreement FRF.2. The NNI interface is concerned
with the transfer of C-plane and U-plane information between two
network nodes belonging to two different Frame Relay networks. Such
frames are automatically recognized by the analyzer and correctly
displayed.
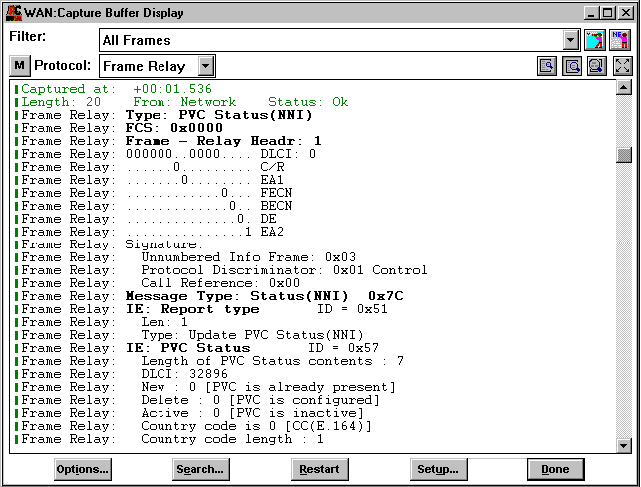
NNI PVC
decode
FRF.3 provides multiprotocol
encapsulation over Frame Relay within an ANSI T1.618 frame. The
structure of such a Frame Relay frame is as follows:
8 |
7 |
6 |
5 |
4 |
3 |
2 |
1 |
Octet
|
Flag (7E hex )
|
1 |
T1.618 Address (including
10-bit DLCI) |
2 3
|
Q.922 Control (UI or I frame)
|
4 |
Optional Pad (0x00)
|
5 |
NLPID |
6 |
Data . .
|
7
|
Frame Check Sequence
|
n-2 n-1
|
Flag (7E hex)
|
n
|
FRF.3 frame
structure
The NLPID (Network Level Protocol ID)
field designates what encapsulation or what protocol follows. The
following diagram details the possible values for NLPID and the
protocols which are designated by each value:
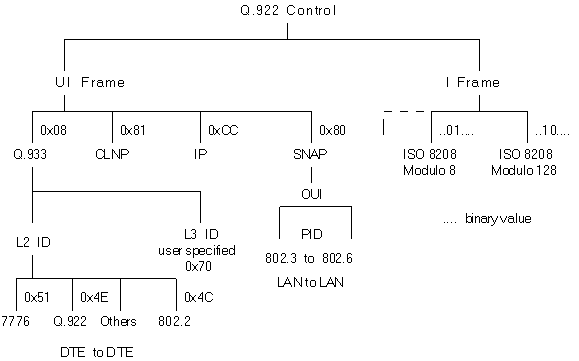
Multiprotocol encapsulation over
Frame Relay
For example, a value of 0xCC indicates
an encapsulated IP frame.
FRF.4 is Frame Relay switched virtual
connection user-to-network interface agreement. It applies using
equipment attached to a non-ISDN Frame Relay network or to an ISDN
network using only case A.
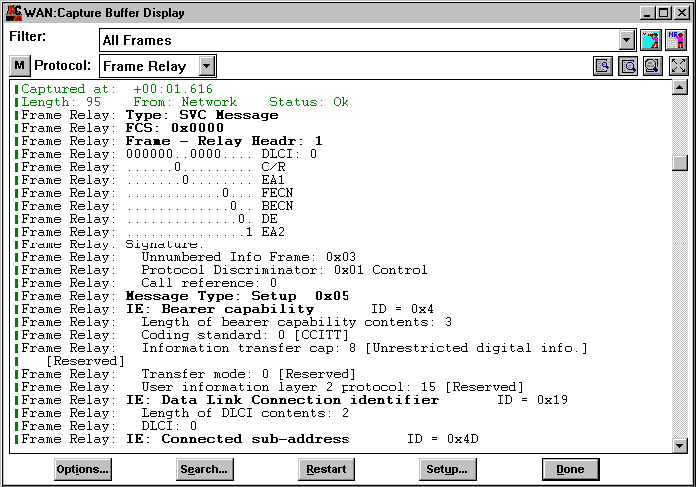
UNI SVC
decode
Following is a list of valid SVC message
types:
- Call proceeding.
- Connect.
- Connect Acknowledge.
- Disconnect.
- Progress.
- Release.
- Release complete.
- Setup.
- Status.
- Status enquiry.
FRF.5
FRF.5 defines Network
internetworking connecting Frame Relay over ATM. Refer to (Frame Relay
over ATM) for more details.
FRF.8
FRF.8 defines Service
internetworking connecting Frame Relay over ATM. Refer to (Frame
Relay over ATM) for more details.
DCP (FRF.9)
FRF. 9 RFC
1661
Your analyzer also supports the decoding
of Network-to-Network (NNI) SVC frames according to the Frame Relay
Forum implementation agreement FRF.10. This implementation agreement
applies to SVCs over Frame Relay NNIs and to SPVCs. It is applicable
at NNIs whether both networks are private, both are public or one is
private and the other public. Such frames are automatically
recognized by the analyzer and correctly displayed.
FRF.12
FRF.12 is the Frame Relay Fragmentation
Implementation Agreement. Fragmentation queuing reduces both delay
and delay variation in Frame Relay networks by dividing large data
packets into smaller packets and then reassembling the data into the
original frames at the destination. This ability is particularly
relevant to users who wish to combine voice and other time-sensitive
applications, such as SNA mission-critical applications, with
non-time-sensitive applications or other data on a single Permanent
Virtual Circuit (PVC). The main benefit of fragmentation is the
ability to utilize common User to Network Interface (UNI) access
lines or Network to Network Interface (NNI) lines and/or PVCs for
communications combining large data frames and real-time protocols.
Fragmenting frames enhances the utility and
uniformity of Frame Relay networks, reducing delay and delay
variation while upgrading application responsiveness, quality and
reliability. As a result, multiple types of traffic, such as voice,
fax and data, can be transparently combined on a single UNI, NNI
and/or PVC.
The Fragmentation Implementation Agreement
provides for transmission of Frame Relay Data Terminal Equipment
(DTE) and Data Communications Equipment (DCE) with the ability to
fragment long data frames into a sequence of shorter frames that are
then reassembled into the original frame by the receiving peer DTE
or DCE. Frame fragmentation is necessary to control delay and delay
variation when real-time traffic, such as voice, is carried across
the same interfaces as data traffic. Fragmentation enables the
interleaving of delay-sensitive traffic on one PVC with fragments of
long data frames on another PVC using the same interface.
FRF.12 supports three fragmentation
applications:
- Locally across a Frame Relay UNI interface
between the DTE/DCE peers.
- Locally across a Frame Relay NNI interface
between DCE peers.
- End-to-End between two Frame Relay DTEs
interconnected by one or more Frame Relay networks.
When used end-to-end, the fragmentation
procedure is transparent to Frame Relay network(s) between the
transmitting and receiving DTEs.
FREther
FREther is a variant of Frame Relay
which is comprised of the Frame Relay header followed by the
EtherType field. This is an additional form of encapsulation over
Frame Relay which is used by some customers.
Timeplex
(BRE2)
BRE (Bridge Relay Encapsulation) is a
proprietary Ascom Timeplex protocol that extends bridging across WAN
links by means of encapsulation. BRE2 is an improved form of the
standard, providing better performance due to the fact that it sits
directly on the link layer protocol, requires less configuration and
provides its own routing protocol. BRE2 was deployed in 4.0 router
software and is available in all 4.x and 5.x versions of the
software.
The format of the BRE2 frame is as
follows:
Frame Type
|
F |
Priority
|
Source BRE Bridge No.
|
|
Source Bridge Domain ID =1
|
VLAN ID
|
SRB #
|
Remainder of BRE2 Header
|
7 bytes in unfragmented format
|
12 bytes in fragmented format
|
|
Data
|
|
BRE2 frame
format
If F is 0 than the frame is unfragmented
and the BRE2 header is 17 bytes long. If F is 1, the frame is
fragmented and the BRE2 header is 22 bytes long. SRB # is the Source
Route Bridge Number (4 bits).
Cascade
In order to provide a Frame Relay
service, Regional Bell Operating Companies (RBOCs) deploy Cascade
switches in multiple LATAs and interconnect them to provide service
to customers across the LATAs, as well as manage the switches in
multiple LATAs from a single network management station.
The trunk header format for the Cascade
STDX family of switches conform to ANSI T1.618-1991 ISDN Core
Aspects of Frame Protocol for use with Frame Relay Bearer Service.
The Cascade trunk header format is as
shown below:
|
|
|
|
|
R |
C/R |
Version
|
Reserved
|
ODE |
DE |
BECN |
FECN |
VC priority
|
Channel ID |
User
Management data depending
|
Cascade trunk header
format
For management data, a 5th byte contains
information concerning the type of PDU information to follow. Values
are as follows:
0 |
Call request
PDU |
1 |
Confirmation
PDU |
2 |
Rejected
PDU |
3 |
Clear
PDU |
4 |
Disrupt
PDU |
5 |
Hello
PDU |
6 |
Hello Acknowledgment
PDU |
7 |
Defined Path Hello
PDU |
8 |
Defined Path Hello
Acknowledgment PDU |
LAPF
The purpose of LAPF is to convey data
link service data units between DL-service users in the U-plane for
frame mode bearer services across the ISDN user-network interface on
B-, D- or H-channels. Frame mode bearer connections are established
using either procedures specified in Recommendation Q.933 [3] or
(for permanent virtual circuits) by subscription. LAPF uses a
physical layer service, and allows for statistical multiplexing of
one or more frame mode bearer connections over a single ISDN B-, D-
or H-channel by use of LAPF and compatible HDLC procedures.
The format of the header is shown in the
following illustration:
|
8 |
7 |
6 |
5 |
4 |
3 |
2 |
1 |
Default address field format
(2 octets) |
Upper DLCI |
C/R |
EA 0 |
Lower DLCI |
FECN (Note) |
BECN (Note) |
DE (Note) |
EA 1 |
LAPF address
format
Control field
bits (Modulo 128) |
8 |
7 |
6 |
5 |
4 |
3 |
2 |
1 |
|
I Format |
N(S) |
0 |
Octet 4 (Note) |
N(R) |
P/F |
Octet 5 |
S Format |
X |
X |
X |
X |
Su |
Su |
0 |
1 |
Octet 4 |
N(R) |
P/F |
Octet 5 |
U Format |
M |
M |
M |
P/F |
M |
M |
1 |
1 |
Octet 4 |
LAPF format
search ][ protocols
by family ][ index of
protocols
|